Sleep and Arousal
- Shamsi Mumtahina momo
- Feb 2, 2023
- 9 min read
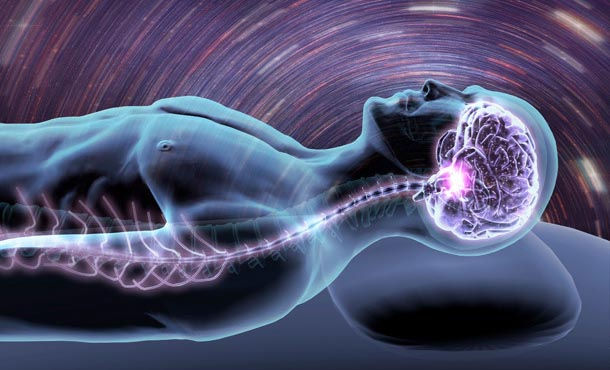
Sleep is an important part of your daily routine—you spend about one-third of your time doing it. Arousal is a state of awareness of the external world. The counterpart of arousal is sleep, when an individual continues to receive external stimuli but is not aware of them.It is controlled by several centers in the brainstem and cerebrum.A diffuse network of neurons called the reticular formation containing over 90 separate clusters of cell bodies, is present at the core of the brainstem. A part of the reticular formation, the reticular activating system (RAS), regulates sleep and arousal. Acting as a sensory filter, the RAS selects which information reaches the cerebral cortex, and the more information the cortex receives, the more alert a person is. Also, sleep and wakefulness are regulated by specific parts of the brainstem: The pons and medulla contain centers that cause sleep when stimulated, and the midbrain has a center that causes arousal. Serotonin may act here as a neurotransmitter.
Sleep Stages

There are two main stages of sleep known as rapid eye movement (REM) and non-rapid eye movement sleep (NREM) or slow-wave sleep (SWS). During REM sleep, the brain activity is similar to that of an awake person, whereas NREM sleep is highlighted by periods of slow waves and synchronized neuron activity.
Stage 1 non-REM sleep is the changeover from wakefulness to sleep. During this short period (lasting several minutes) of relatively light sleep, your heartbeat, breathing, and eye movements slow, and your muscles relax with occasional twitches. Your brain waves begin to slow from their daytime wakefulness patterns.
Stage 2 non-REM sleep is a period of light sleep before you enter deeper sleep. Your heartbeat and breathing slow, and muscles relax even further. Your body temperature drops and eye movements stop. Brain wave activity slows but is marked by brief bursts of electrical activity. You spend more of your repeated sleep cycles in stage 2 sleep than in other sleep stages.
Stage 3 non-REM sleep is the period of deep sleep that you need to feel refreshed in the morning. It occurs in longer periods during the first half of the night. Your heartbeat and breathing slow to their lowest levels during sleep. Your muscles are relaxed and it may be difficult to awaken you. Brain waves become even slower.
Stage 4 REM sleep first occurs about 90 minutes after falling asleep. Your eyes move rapidly from side to side behind closed eyelids. Mixed frequency brain wave activity becomes closer to that seen in wakefulness. Your breathing becomes faster and irregular, and your heart rate and blood pressure increase to near waking levels. Most of your dreaming occurs during REM sleep, although some can also occur in non-REM sleep. Your arm and leg muscles become temporarily paralyzed, which prevents you from acting out your dreams. As you age, you sleep less of your time in REM sleep. Memory consolidation most likely requires both non-REM and REM sleep.
Neurological regulation of sleep:
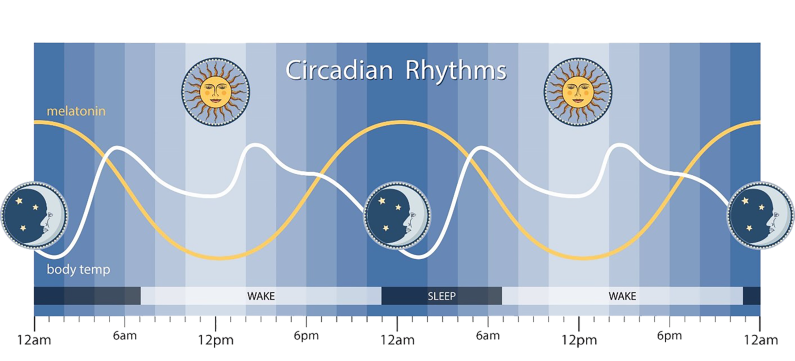
The timing of sleep is regulated by the circadian rhythm, which is the internal clock of the body that dictates the urge to sleep according to the surrounding environment and light cues.The circadian rhythm is controlled by the suprachiasmatic nucleus (SCN) in the hypothalamus, which processes light signals from the optic nerve and triggers the release of certain neurotransmitters. The SCN monitors changes in body temperature, the production of cortisol hormone, as well as the release of melatonin, all of which have an effect on sleep. Acetylcholine is an important neurotransmitter in the regulation of sleep, particularly within the pons and the basal forebrain of the brain, as it helps to increase cortical arousal. Other neurotransmitters involved in the regulation of sleep, include gamma aminobutyric acid (GABA), which promotes sleep, as well as norepinephrine, serotonin, histamine and orexin, which increase alertness and hinder sleep.
Sleep functions:
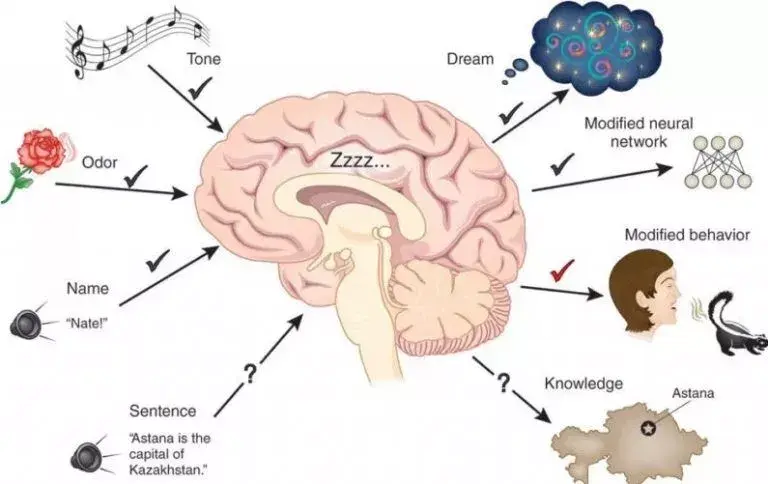
Sleep eases pain and anxiety in the brain
The neuroscience of sleep helps explain how sleep helps us learn and forget. It also sheds light on sleep’s pain-relieving and anti-anxiety effect.For example, a study published last year found that a brain area associated with pain sensitivity (called the somatosensory cortex) is hyperactive in sleep-deprived participants. The findings suggested that not getting enough sleep interferes with the brain’s pain-processing neural circuits.Additionally, the same study found that activity in the nucleus accumbens area of the brain had dropped after a sleepless night. The nucleus accumbens releases the neurotransmitter dopamine, which heightens feelings of pleasure and decreases sensations of pain.“Sleep loss not only amplifies the pain-sensing regions in the brain but blocks the natural analgesia centers, too,” explains Matthew Walker, a professor of neuroscience and psychology at the University of California in Berkeley.The team also found that the brain’s insula, which evaluates pain signals and prepares the pain response, was also underactive in sleep-deprived people.Sleeplessness interferes with this “critical neural system that assesses and categorizes the pain signals and allows the body’s natural painkillers to come to the rescue.”
Memory processing
According to Plihal & Born, sleep generally increases recalling of previous learning and experiences, and its benefit depends on the phase of sleep and the type of memory.[134] For example, studies based on declarative and procedural memory tasks applied over early and late nocturnal sleep, as well as wakefulness controlled conditions, have been shown that declarative memory improves more during early sleep (dominated by SWS) while procedural memory during late sleep (dominated by REM sleep).Regarding to declarative memory, the functional role of SWS has been associated with hippocampal replays of previously encoded neural patterns that seem to facilitate long-term memories consolidation. This assumption is based on the active system consolidation hypothesis, which states that repeated reactivations of newly encoded information in hippocampus during slow oscillations in NREM sleep mediate the stabilization and gradual integration of declarative memory with pre-existing knowledge networks on the cortical level. It assumes the hippocampus might hold information only temporarily and in fast-learning rate, whereas the neocortex is related to long-term storage and slow-learning rate.This dialogue between hippocampus and neocortex occurs in parallel with hippocampal sharp-wave ripples and thalamocortical spindles, synchrony that drives the formation of spindle-ripple event which seems to be a prerequisite for the formation of long-term memories.Reactivation of memory also occurs during wakefulness and its function is associated with serving to update the reactivated memory with new encoded information, whereas reactivations during SWS are presented as crucial for memory stabilization. Based on targeted memory reactivation (TMR) experiments that use associated memory cues to triggering memory traces during sleep, several studies have been reassuring the importance of nocturnal reactivations for the formation of persistent memories in neocortical networks, as well as highlighting the possibility of increasing people’s memory performance at declarative recalls.Furthermore, nocturnal reactivation seems to share the same neural oscillatory patterns as reactivation during wakefulness, processes which might be coordinated by theta activity. During wakefulness, theta oscillations have been often related to successful performance in memory tasks, and cued memory reactivations during sleep have been showing that theta activity is significantly stronger in subsequent recognition of cued stimuli as compared to uncured ones, possibly indicating a strengthening of memory traces and lexical integration by cuing during sleep. However, the beneficial effect of TMR for memory consolidation seems to occur only if the cued memories can be related to prior knowledge.
Other studies have been also looking at the specific effects of different stages of sleep on different types of memory. For example, it has been found that sleep deprivation does not significantly affect recognition of faces, but can produce a significant impairment of temporal memory (discriminating which face belonged to which set shown). Sleep deprivation was also found to increase beliefs of being correct, especially if they were wrong. Another study reported that the performance on free recall of a list of nouns is significantly worse when sleep deprived (an average of 2.8 ± 2 words) compared to having a normal night of sleep (4.7 ± 4 words). These results reinforce the role of sleep on declarative memory formation. This has been further confirmed by observations of low metabolic activity in the prefrontal cortex and temporal and parietal lobes for the temporal learning and verbal learning tasks respectively. Data analysis has also shown that the neural assemblies during SWS correlated significantly more with templates than during waking hours or REM sleep. Also, post-learning, post-SWS reverberations lasted 48 hours, much longer than the duration of novel object learning (1 hour), indicating long term potentiation. Moreover, observations include the importance of napping: improved performance in some kinds of tasks after a 1-hour afternoon nap; studies of performance of shift workers, showing that an equal number of hours of sleep in the day is not the same as in the night. Current research studies look at the molecular and physiological basis of memory consolidation during sleep. These, along with studies of genes that may play a role in this phenomenon, together promise to give a more complete picture of the role of sleep in memory.
Deep sleep relieves anxiety in key brain region
As to the anxiolytic effects of sleep, functional MRI scans and polysomnograms have shown that the medial prefrontal cortex in the brain is key. This region deactivated after a sleepless night in some studies co-led by Prof. Walker.Previous research suggests that the medial prefrontal cortex helps calm anxiety and reduce stress levels. In Prof. Walker’s research, other regions associated with processing emotions were hyperactive in sleep-deprived patients.“Without sleep,” Prof. Walker explains, “it’s almost as if the brain is too heavy on the emotional accelerator pedal, without enough brake.” A sleepless night caused anxiety levels to spike by up to 30% in their study, report the scientists.Furthermore, the study found that anxiety levels dropped after a full night of sleep and that this decrease was even sharper in participants who spent more time in the deep non-REM stage of sleep.
Sleep helps the brain learn and stay flexible
In the first study, the experimenters tampered with the study participants’ deep, non-REM sleep stage after asking them to learn a new set of movements. The scientists monitored the participants’ brain activity — their motor cortex, specifically — throughout the study.The team — led by Switzerland-based scientists — found that a restless deep sleep resulted in a visibly reduced learning efficiency. The researchers’ explained that their results hinged on the brain’s synapses and their roles in learning.Synapses are microscopic connections between neurons that, together with brain chemicals, or neurotransmitters, facilitate the passing of electrical impulses from one neuron to another. During the day, synapses switch on in response to the stimuli that the brain receives from the environment.But during sleep, the activity of these synapses goes back to normal. Without this restorative period, they stay excited at their peak activity for too long.This interferes with the brain’s neuroplasticity, that is, its ability to rewire itself and create new connections between neurons. Neuroplasticity enables the brain to ‘pick up’ new skills, change and adapt to its environment stimuli, and ultimately learn new things.
Waste clearance from the brain
During sleep, metabolic waste products, such as immunoglobulins, protein fragments or intact proteins like beta-amyloid, may be cleared from the interstitial via a glymphatic system of lymph-like channels coursing along perivascular spaces and the astrocyte network of the brain.According to this model, hollow tubes between the blood vessels and astrocytes act like a spillway allowing drainage of cerebrospinal fluid carrying wastes out of the brain into systemic blood. Such mechanisms, which remain under preliminary research as of 2017, indicate potential ways in which sleep is a regulated maintenance period for brain immune functions and clearance of beta-amyloid, a risk factor for Alzheimer's disease.
Endocrine function
The secretion of many hormones is affected by sleep-wake cycles. For example, melatonin, a hormonal timekeeper, is considered a strongly circadian hormone, whose secretion increases at dim light and peaks during nocturnal sleep, diminishing with bright light to the eyes. In some organisms melatonin secretion depends on sleep, but in humans it is independent of sleep and depends only on light level. Of course, in humans as well as other animals, such a hormone may facilitate coordination of sleep onset. Similarly, cortisol and thyroid stimulating hormone (TSH) are strongly circadian and diurnal hormones, mostly independent of sleep. In contrast, other hormones like growth hormone (GH) & prolactin are critically sleep-dependent, and are suppressed in the absence of sleep. GH has maximum increase during SWS while prolactin is secreted early after sleep onset and rises through the night. In some hormones whose secretion is controlled by light level, sleep seems to increase secretion. Almost in all cases, sleep deprivation has detrimental effects. For example, cortisol, which is essential for metabolism (it is so important that animals can die within a week of its deficiency) and affects the ability to withstand noxious stimuli, is increased by waking and during REM sleep. Similarly, TSH increases during nocturnal sleep and decreases with prolonged periods of reduced sleep, but increases during total acute sleep deprivation.
Because hormones play a major role in energy balance and metabolism, and sleep plays a critical role in the timing and amplitude of their secretion, sleep has a sizable effect on metabolism. This could explain some of the early theories of sleep function that predicted that sleep has a metabolic regulation role.
Renormalizing the synaptic strength
Sleep can also serve to weaken synaptic connections that were acquired over the course of the day but which are not essential to optimal functioning. In doing so, the resource demands can be lessened, since the upkeep and strengthening of synaptic connections constitutes a large portion of energy consumption by the brain and tax other cellular mechanisms such as protein synthesis for new channels. Without a mechanism like this taking place during sleep, the metabolic needs of the brain would increase over repeated exposure to daily synaptic strengthening, up to a point where the strains become excessive or untenable.
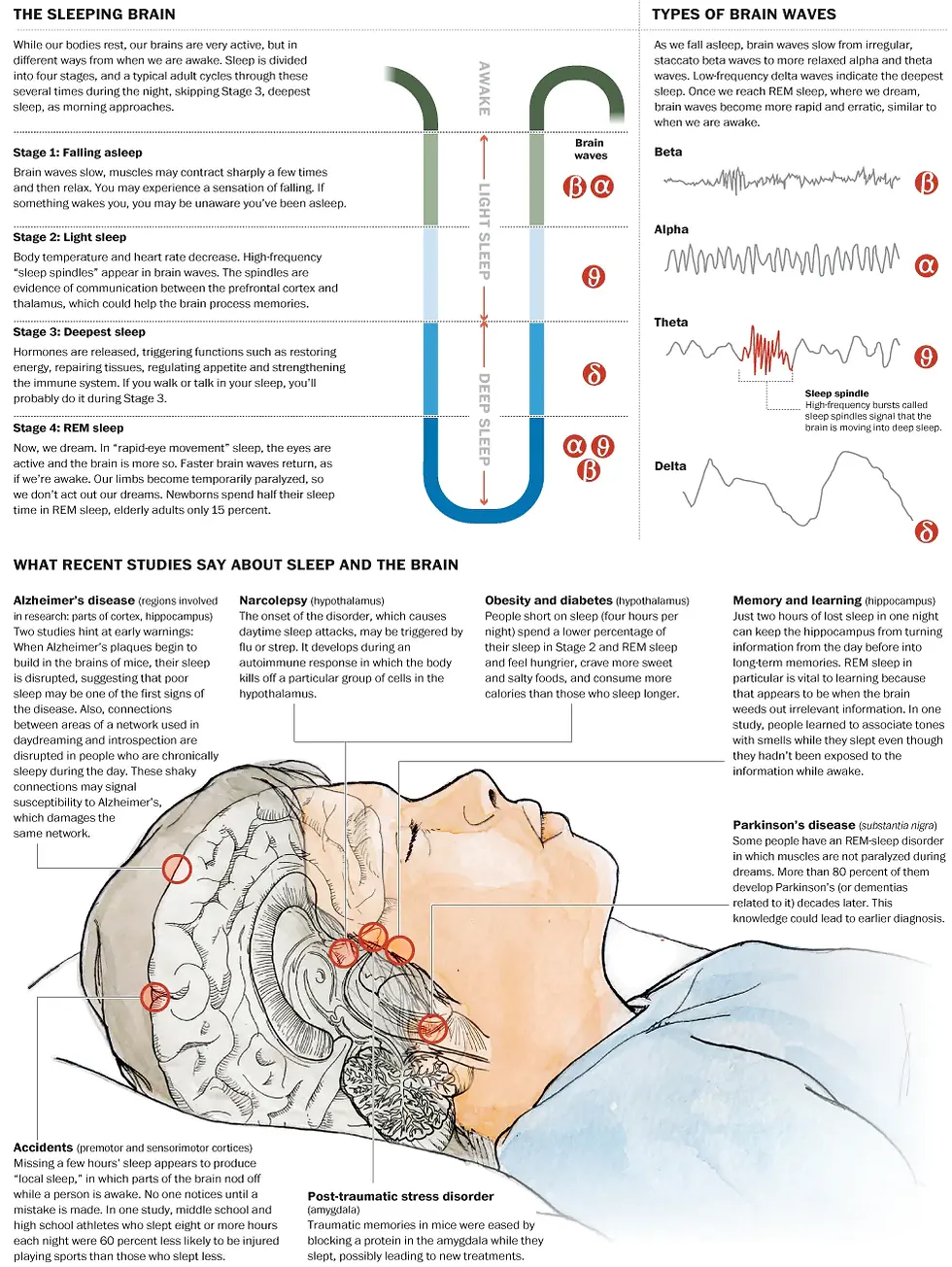
Lack of sleep can cause several health problems. One in five U.S. adults shows signs of chronic sleep deprivation, and a shortage of sleep has been linked to health problems as different as diabetes and Alzheimer’s disease. Recent studies have found some interesting connections between illness and what is happening in our brains as we snooze.
References:
留言